How merging black holes could reveal the nature of dark matter
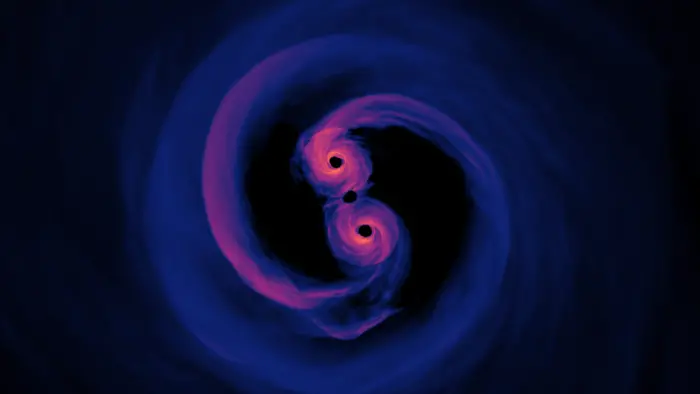
A screenshot from a simulation shows two merging supermassive black holes. Credit: NASA’s Goddard Space Flight Center/Scott Noble; simulation data, d’Ascoli et al. 2018
We see evidence for merging supermassive black holes everywhere we look. One problem: We’re not exactly sure how they manage to do it. Recently, a team of astronomers has proposed that a particular form of dark matter may be the key to unlocking this cosmic mystery.
Supermassive black holes are the largest black holes in the universe. They weigh anywhere from a few hundred thousand times to a few hundred billion times the mass of the Sun. Almost every galaxy in the universe hosts such a giant black hole in its core.
Astronomers believe these black holes grew to their enormous proportions over the course of billions of years, from a combination feeding on any material that happens to wander too close and from merging with other giant black holes. Last year, a team used an observational technique known as a pulsar timing array to pick up a “background hum” of gravitational waves consistent with a large population of merging supermassive black holes. These gravitational waves are emitted as the black holes spiral in toward each other during the merger event.
Related: The Great Hum: Scientists finally detect continuous gravitational waves rippling across space-time
But in between those two scales, astronomers don’t know how the black holes lose energy. When they are separated by roughly a parsec — 3.26 light-years — there isn’t enough material to serve as a reservoir for energy, and the gravitational waves aren’t strong enough to do it on their own. Hence the problem.
However, astronomers aren’t exactly sure how the merger process unfolds. What’s particularly challenging is an issue known as the final parsec problem. To get two black holes to merge, they have to come close to each other. And to come close to each other, they have to lose orbital energy. At large distances, the black holes can lose orbital energy through a variety of means, such as interacting with the gas or stars in a galaxy. By the time the black holes are a short distance apart, just a handful of light-years, all the material has been cleared away, but by then the emission of gravitational waves can pull energy out of the system, allowing the black holes to collide.
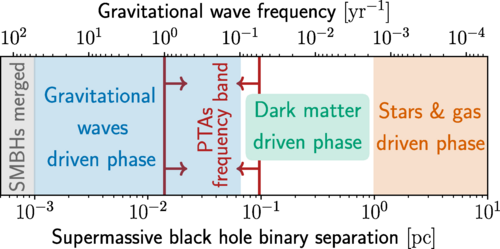
Self-interacting dark matter
A new paper published July 9 in Physical Review Letters offers a potential solution to this problem. The authors’ solution involves dark matter, the invisible matter that appears to dominate the mass of galaxies. While there is a substantial amount of circumstantial evidence for the existence of dark matter, such as the rotation rate of galaxies and the growth of large structures in the universe, we do not yet know the identity of the dark matter particle. All we know is that dark matter does not interact with light. It only interacts with the rest of the universe through gravity.
The simplest model for dark matter assumes that it is collisionless, which means that dark matter does not interact with itself. But there are some models of dark matter where it can weakly interact with itself. These models are known, appropriately enough, as self-interacting dark matter. Astronomers proposed this model of dark matter decades ago as a way to solve some of the deficiencies with the collisionless dark matter idea. Most importantly, collisionless dark matter has difficulty producing the observed number of dwarf satellite galaxies and matching the observed densities in the cores of galaxies.
“The possibility that dark matter particles interact with each other is an assumption that we made, an extra ingredient that not all dark matter models contain,” said study co-author Gonzalo Alonso-Álvarez, a postdoctoral fellow in the Department of Physics at the University of Toronto and the Department of Physics and Trottier Space Institute at McGill University, in a press release. “Our argument is that only models with that ingredient can solve the final parsec problem.”
The astronomers behind the study found that when they switched out collisionless dark matter for self-interacting dark matter in their models, the final parsec problem wasn’t a problem anymore. Supermassive black holes are thought to merge as the last stage in the merger of two galaxies. As the supermassive black holes enter the core of the resulting single galaxy, they encounter greater densities of dark matter. The black holes interact with the dark matter through gravity — just as they do with gas and stars when they are farther apart — which siphons energy from the momentum of the black hole into the dark matter particles. With collisionless dark matter, the dark matter particles pick up this extra energy and then simply leave. But with self-interacting dark matter, the extra energy added to the particles just goes into more interactions.
This allows the dark matter to act as a reservoir that can absorb the kinetic energy of the black holes as they come closer together. With this extra reservoir available, the supermassive black holes quickly close the final parsec and meet in their final gravitational embrace.
Looking for confirmation
The researchers predict that in such a scenario, the resulting gravitational waves should be shifted slightly from the expected case with collisionless dark matter. And this is exactly what astronomers have seen with pulsar timing arrays.
“A prediction of our proposal is that the spectrum of gravitational waves observed by pulsar timing arrays should be softened [lower power] at low frequencies,” said James Cline, study co-author and Professor at McGill University and the CERN Theoretical Physics Department in Switzerland. “The current data already hint at this behavior, and new data may be able to confirm it in the next few years.”
While it’s not a confirmed detection of dark matter or a confirmed solution to the final parsec problem, this study does show that dark matter may be more complicated than we naively assume, and it may play a significant role in the evolution of black holes.
And, in turn, black holes may help us reveal the true nature of dark matter.
“Our work is a new way to help us understand the particle nature of dark matter,” said Alonso-Álvarez. “We found that the evolution of black hole orbits is very sensitive to the microphysics of dark matter and that means we can use observations of supermassive black hole mergers to better understand these particles.”
Post Comment